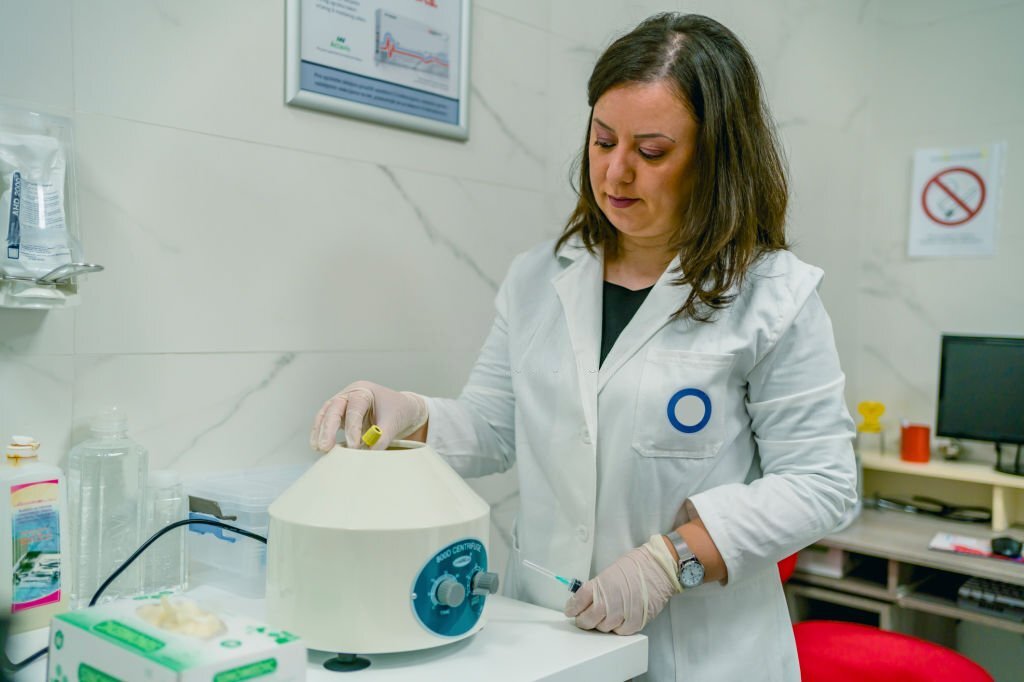
Related Articles
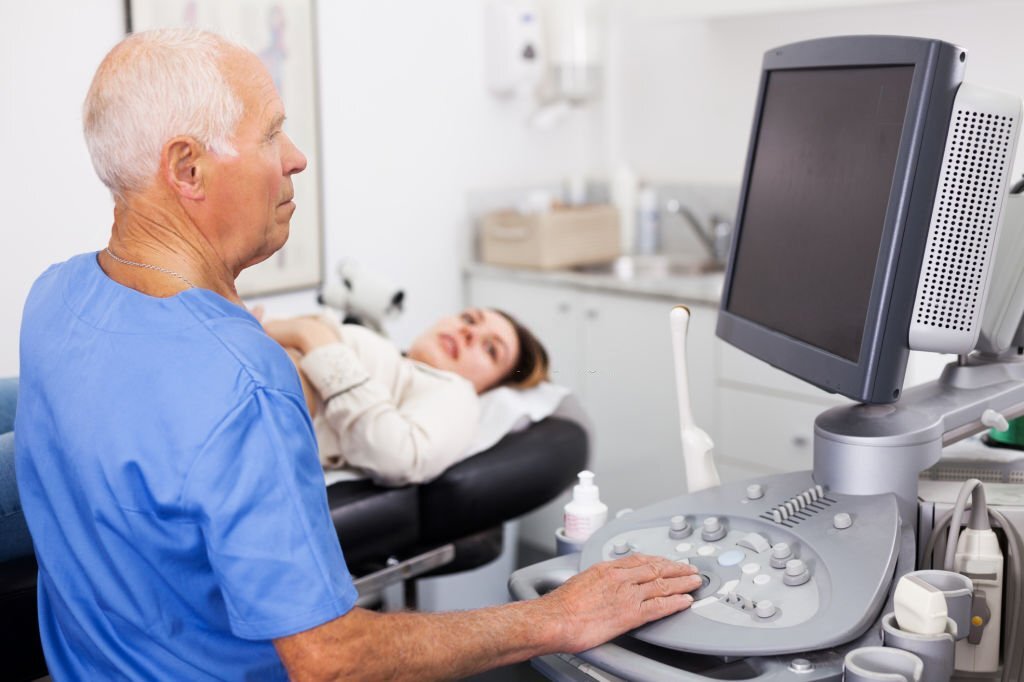
Resources
AMBAR Study Demonstrates the Feasibility of Two Plasma Exchange Modalities for Alzheimer's Treatment
May 2, 2023
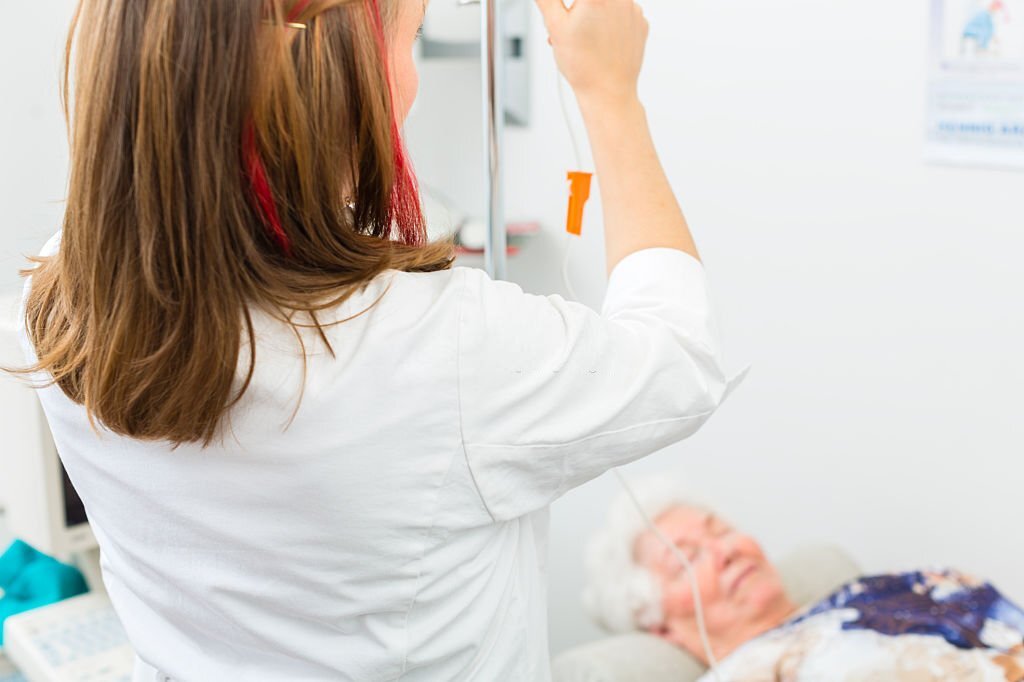
Resources
Intermittent Heterochronic Plasma Exchange as a Modality for Delaying Cellular Senescence—A Hypothesis
May 2, 2023
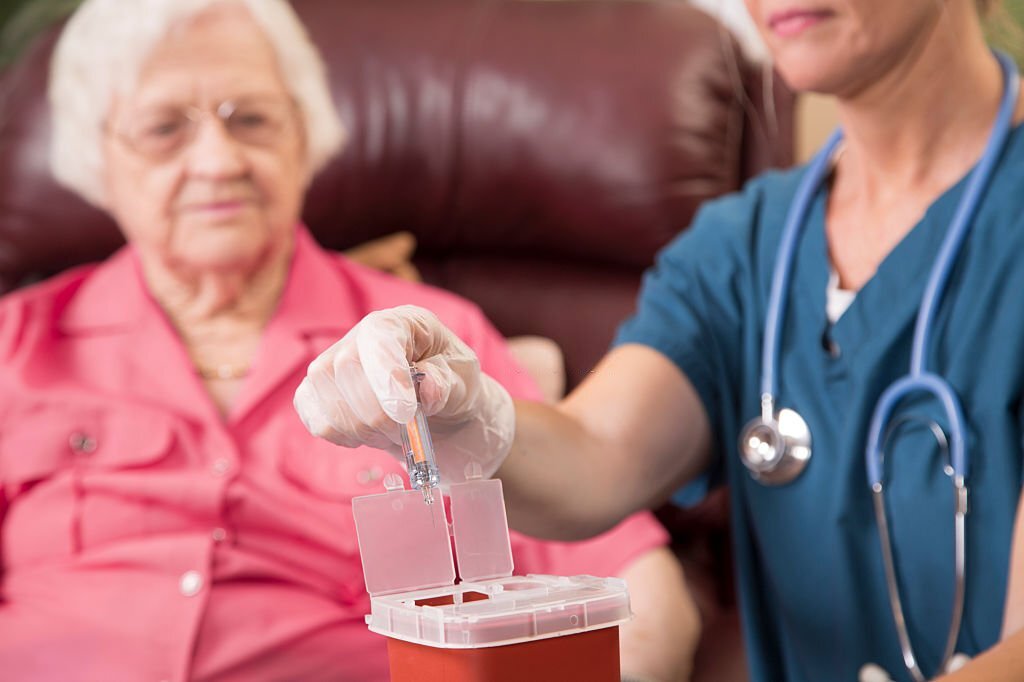
Resources
Therapeutic plasma exchange (TPE) and blood products – Implications for longevity and disease
May 2, 2023
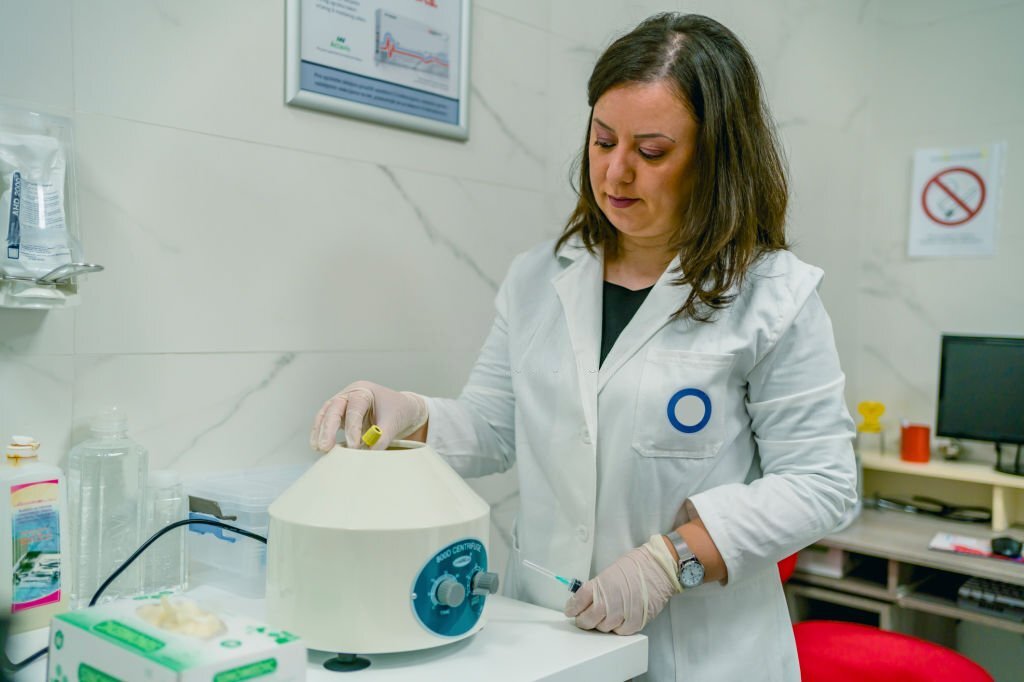
Resources
Novel Approach to Attenuate Age-Elevated Blood Factors through Repositioning Plasmapheresis
May 2, 2023

May 2, 2023
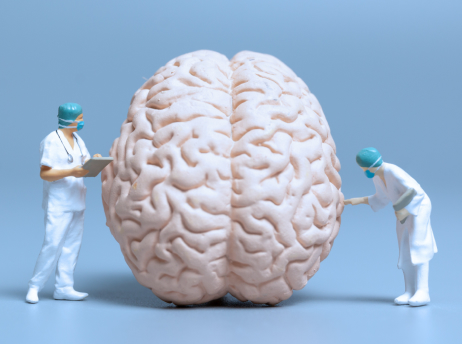
May 2, 2023